Imaging and characterization of dental structure using optical coherence tomography
Optical probing of dental structure has thus far been primarily limited to visible and ultravio- let wavelengths' where scattering dominates the propagation of light. Optical coherence to- mography (OCT) techniques, however, have demonstrated that light in the near-infrared region of the spectrum can often provide higher resolution interior imaging of biologi- cal tissue~.~-5 Here, we use OCT to obtain high-resolution interior images and investi- gate the optical properties of normal and dis- eased.
Our experiments were carried out in a com- pact fiber-based OCT apparatus operating at 830 nm and 1280 nm wth longitudinal and transverse resolutions of 15 pm and powers of 1 mW. All of our studies were conducted in vitro using extracted human teeth preserved in a thymol solution. Depth scanning was achieved electronically via piezoelectric optical path length modulation in the reference arm of the interferometer. For these experiments, a maximum depth of 3.11 mm (free space) was used. Transverse scanning (up to 1 cm) was accomplished by automatic bending of the top of the optical fiber behind the focusing lens. The operation of the OCT scanner was com- pletely automated ahd controlled by a personal computer. Single transverse scans were accom- plished in 25 s. Three-dimensional scanning was accomplished by automated rastering of the fiber.
dental laser tips
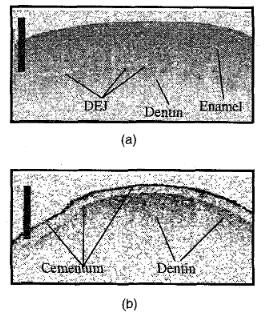
Figure l(a) displays an OCT distal-mesial scan taken at 1280 nm ofthe buccal surface of a normal bicuspid. Both the enamel (the upper right band) and dentin (the lower bright band) are clearly visible in the scan. The dark region separating the enamel and dentin is the dentin- enamel junction (DEJ), a thin region that de- fines the interface. Although we are able to image through the entire 1-mm enamel layer, the effective optical imaging depth in dentin is reduced to < 100 pm owing to increased scat- tering from microstructural tubules. Figure l(b) displays the buccal surface of the root of the same tooth. The cementum outer layer (100 pm) is manifested as the bright surface reflection and subsurface dark band in the scan. Dentin is visible just below the cemen- tum layer.
Dental pathologies are shown in Figs. 2(a) and 2(b), where we display the OCT mesial- distal scan of a subsurface carious lesion [Fig. 2(a)] and a distal-proximal scan at the edge of CTuL6 Fig. 1. (a) OCT distal-mesial scan of the facial enamel of a normal biscupid. Enamel, dentin, and the dentin-enamel junction (DEJ) are clearly visible. The scale bar is 1 mm (horizontal and vertical) and is corrected for the index of refraction of the enamel in the vertical direction. (b) Distal-mesial scan of the facial root of a nor- mal bicuspid. A thin cementum layer is visible over the dentin.
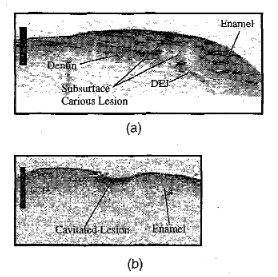
a cavitated lesion [Fig. 2(b)]. In the case of Fig. 2(a), the subsurface structure and enhanced scattering near the surface are consistent with demineralization and subsequent formation of microstructural erosion of the enamel. En- hanced scattering is also evident in Fig. 2(b) below the surface of the cavitated lesion. The optical properties of both enamel and dentin are partially determined by their micro- structure. Enamel consists of protein-bound hydroxyapetite prisms approximately 4 pm in diameter and 1-4 mm in length whose orien- tation varies with position on the tooth. Den- tin consists of a mineral collagen framework evidencing a fluid-filled tubular microstruc- ture. Thus penetration and scattering depend upon the relative direction of irradiation. We will present measurements of scattering in dentin and enamel as well as a comparison of dental OCT images with optical microscopy. *Institute of Applied Physics of the Russian Academy of Sciences, Nizhny Novgorod 603600, Russia.
Tom Collier, Colin Smithpeter, Brett Bowman, Rebekah Drezek, Mike Descour,* Rebecca Richards-Kortum, Biomedical Engineering Program, Department of Electrical and Computer Engineering, University of Texas, Austin, Texas 78731 Recently confocal microscopy has been used to obtain real-time images of skin in vivo with sufficient contrast and resolution to measure nuclear and cytoplasmic diameters.' This tech- nique could be useful for detection of pathol- ogy in internal organs given a fiber-optic in- strument with similar sensitivity and resolution. The principle difficulties associated with this are achieving the necessary resolution (1-3 pm) and rejecting the specular reflections produced at the fiber ends. Two approaches to this problem have been presented; in both a fiber-optic bundle is coupled to a confocal mi- croscope. GmitroZ developed a fiber-optic flu- orescence confocal microscope with 7-pm resolution; specular reflection was avoided by detecting at the fluorescence wavelength. Pho- tobleaching may limit the utility of this system for imaging relatively weak biological autofluorescence. Wilson3 developed a white- light fiber-optic confocal system to image backscattering with 2-pm resolution. This sys- tem used index matching and angle lapping to reduce specular reflections; images of semi- conductors and teeth were acquired. We have developed a prototype fiber-optic confocal mi- croscope to image tissue with 5-pm lateral resolution at 15 frames per sec in vivo; index matching reduces specular reflections. An ex- isting confocal microscope was not required as the entire system was constructed with com- mercially available items.